37 9.2 Solution Stoichiometry
Water is by far the most important liquid solvent, partly because it is plentiful and partly because of its unique properties. In your body, in other living systems, and in the outside environment a tremendous number of reactions take place in aqueous
There are three important classes of reactions which occur in
- Precipitation reactions are useful for detecting the presence of various
ions and for determining the concentrations ofsolutions.
- Acid-
base reactions and redox reactions are similar in that something is being transferred from one species to another.
- Acid-base reactions involve proton transfers, while redox reactions involve electron transfers.
- Redox reactions are somewhat more complicated, though, because proton transfers and other bond-making and bond-breaking processes occur at the same time as electron transfer.
In Binary Ionic Compounds and Their Properties we point out that when an ionic compound dissolves in water, the positive and negative
originally present in the crystal lattice persist in solution. Their ability to move nearly independently through the solution permits them to carry positive or negative electrical charges from one place to another. Hence the solution conducts an electrical current.
Electrolytes
Substances whose solutions conduct electricity are called electrolytes. All soluble ionic compounds are
. They conduct very well because they provide a plentiful supply of
in solution. Some polar covalent compounds are also
. Common examples are HCl, HBr, HI and H2SO4, all of which react with H2O to form large concentrations of
. A solution of HCl, for example, conducts even better than one of NaCl having the same
.
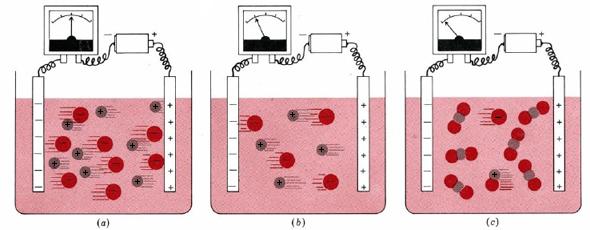
solutions
: (a) 0.1 M NaCl (b) 0.05 M NaCl (c) 0.1 M HgCl2. An electrolyte solution conducts electricity because of the movement of
in the solution (see above). The larger the concentration of ions, the better the solutions conduct.
The effect of the concentration of ions on the electrical current flowing through a solution is illustrated in Figure 11.2.1. Part a of the figure shows what happens when a battery is connected through an electrical meter to two inert metal strips (electrodes) dipping in ethanol. Each cubic decimeter of such a solution contains 0.10 mol NaCl (that is, 0.10 mol Na+ and 0.10 mol Cl–). An electrical current is carried through the solution both by the Na+ ions moving toward the negative electrode and by the Cl– ions which are attracted toward the positive electrode. The dial on the meter indicates the quantity of current.
Figure 1b shows that if we replace the 0.10-M NaCl solution with a 0.05-M NaCl solution, the meter reading falls to about one-half its former value. Halving the
Conductivity measurements reveal that most covalent compounds, if they dissolve in water at all, retain their original molecular structures. Neutral molecules cannot carry electrical charges through the solution, and so no current flows. A substance whose aqueous solution conducts no better than water itself is called a nonelectrolyte. Some examples are oxygen, O2, ethanol, C2H5OH, and sugar, C12H22O11.

Some covalent substances behave as weak electrolytes—their solutions allow only a small current flow, but it is greater than that of the pure solvent. An example is mercury(II) chloride (seen in the Figure above). For a 100-M HgCl2 solution the meter reading shows only about 0.2 percent as much current as for 0.10 M NaCl. A crystal of HgCl2 consists of discrete molecules, like those shown for HgBr2 in Figure 11.2.2. When the solid dissolves, most of these molecules remain intact, but a few dissociate into ions according to the equation
(The double arrows indicate that the ionization proceeds only to a limited extent and an
Conductivity measurements can tell us more than whether a substance is a strong, a weak, or a nonelectrolyte. Consider, for instance, the data in Table 11.2.1 which shows the electrical current conducted through various aqueous
HC2H3O2 (acetic acid) and NH3 (ammonia).
Closer examination of the data for
Substance | Current /mA | Substance | Current /mA |
---|---|---|---|
Pure Water | 1:2 Electrolytes | ||
H2O | 3.69 x 10-4 | Na2SO4 | 2.134 |
Weak Electrolytes
|
Na2CO3 | 2.24 | |
HC2H3O2 | 0.41 | K2CO3 | 2.660 |
NH3 | 0.28 | 2:1 Electrolytes | |
1:1 Electrolytes | MgCl2 | 2.128 | |
NaCl | 1.065 | CaCl2 | 2.239 |
NaI | 1.069 | SrCl2 | 2.290 |
KCl | 1.273 | BaCl2 | 2.312 |
KI | 1,282 | Ba(OH)2 | 4.14 |
AgNO3 | 1.131 | 2:2 Electrolytes | |
HCl | 3.77 | MgSO4 | 2.00 |
HNO3 | 3.75 | CaSO4 | 2.086 |
NaOH | 2.08 | CuSO4 | 1.97 |
KOH | 2.34 | ZnSO4 | 1.97 |
* All measurements refer to a cel1 in which the distance between the electrodes is 1.0 mm and the area of each electrode is 1.0 cm². A potential difference of 1.0 V is applied to produce the tabulated currents.
There is a simple reason for this behavior. Under similar conditions, most
A similar argument applies to
A second, slightly more subtle, conclusion can be drawn from the data in Table 11.2.1. When an electrolyte dissolves, each type of ion makes an independent contribution to the current the solution conducts. This can be seen by comparing NaCl with KCl, and NaI with KI. In each case the compound containing K+ conducts about 0.2 mA more than the one containing Na+. If we apply this observation to Na2CO3 and K2CO3, each of which produces twice as many Na+ or K+
Thus conductivity measurements confirm our statement that each ion exhibits its own characteristic properties in aqueous
At 18°C a 0.001-M
Solution
Referring to Table 6.2 which lists possible
- K+ and H+ and C4+ and three O2–
- K+ and H+ and CO32–
- K+ and HCO3–
Since the current conducted by the solution falls in the range of 1.0 to 1.3 mA characteristic of 1:1 electrolytes, possibility c is the only reasonable choice.
https://youtu.be/2EQznGPZY5A