(2021) First Place: Sage Steppe Post-Fire Recovery Dynamics on Bromus Tectorum, Native Bunchgrasses, and Artemesia Tridentata at Deer Flat National Wildlife Refuge
By Rachel Capezza
ABSTRACT
Sage steppe communities, composed of sagebrush (Artemesia tridentata) and a variety of native grasses, are vital ecosystems for a variety of animal wildlife, including sage grouse, small mammals, and raptors. Anthropogenic disturbances have led to increased fire frequency and the establishment of non-native, invasive plant species which put both plant and animal species within sage steppe communities at risk. Our research focused on the efficacy of recovery efforts at Deer Flat National Wildlife Refuge following the Sage Fire in July 2003. We established two treatment sites denoted as burned and unburned to determine whether recovery efforts in the burned treatment site effectively increased sagebrush density to levels comparable to the unburned treatment site. We also measured comparative foliar cover of both native and nonnative understory plant species, with particular focus on cheatgrass (Bromus tectorum). Statistical analysis of comparative foliar cover of understory species and density of sagebrush yielded mixed results but indicated overall marginal success of recovery efforts in the burned site. Sagebrush density and cheatgrass prevalence remain suboptimal in both burned and unburned sites, suggesting that there is still much work to be done for the recovery and conservation of the sage steppe community at Deer Flat National Wildlife Refuge.
INTRODUCTION
Arid shrublands in the Western United States are ecosystems classified as having little annual precipitation, with particularly hot and dry summer conditions. Sage steppe communities are one such western shrubland, consisting of sagebrush (Artemisia Tridentata), native grasses, and a variety of wildlife including sage grouse (Dumroese et al., 2015), small mammals such as bats (Whiting et al., 2018), and a variety of raptors (Olson et al., 2015) all of which depend on sagebrush either for habitat or food resources. Anthropogenic disturbances to these landscapes, including agricultural overgrazing, plowing, road-building, and recreational activities, have increased fire frequency and severity in these communities. These disturbances have led to a reduction of sagebrush density and the introduction of non-native annual grasses such as cheatgrass (Bromus tectorum) in sage steppe ecosystems (Chambers et al, 2015). Cheatgrass, native to Eurasia, is a ruderal species that is particularly adept at colonizing semi-arid post-fire landscapes such as sage steppe ecosystems (Bradford and Lauenroth, 2006). Furthermore, as cheatgrass is highly flammable, its increased prevalence in sage steppe ecosystems exacerbate fire severity when it occurs, promoting continuous cycles of fire disturbance that threaten sagebrush abundance and inhibit success of its post-fire recovery. Frequency of fire disturbance is also greatly altered, as fire disturbance intervals decrease from approximately every 107 years for unaltered sage steppe communities to every nine years if cheatgrass or other non-native annual grasses have established dominance (Weltz et al., 2014, as cited in Jones et al., 2015). Sage steppe ecosystems characterized by low elevation sites and hot, arid climates, such as the communities which are the focus of this study, are particularly susceptible to fire disturbance and colonization of ruderal species, demonstrating both lowered resistance and resilience in response to disturbance (Chambers et al, 2015). In light of these challenges, reduction, and management of cheatgrass is an important focus of our study, as its colonization post-fire can quickly spread, hindering recovery of native grasses and shrubs (Bates et al., 2011) implicating risks of long-term structural changes to sage steppe communities. Thus, understanding the role that cheatgrass plays in the degradation of sage steppe ecosystems is a vital component to the development of management and recovery strategies for sage steppe ecosystems that are vulnerable or exposed to fire disturbance.
Cheatgrass, an early germinating annual, capitalizes on the cool, mesic conditions typical of sage steppe communities in the late fall or early winter, outcompeting native plants by colonizing and acquiring space through rapid proliferation and limiting nutrient and water resources for the native plant community (Chambers et al, 2015). Furthermore, cheatgrass invasion can result in a shift in the composition and diversity of soil microbiota, altering ecosystem characteristics that encourage their continued colonization while potentially suppressing growth and fitness of native species such as sagebrush and bunchgrasses (Gehring et al, 2016). Understanding the impacts of cheatgrass invasion on soil suitability for native species of sage steppe communities is of fundamental importance when determining management strategies to encourage their recovery and growth. Moreover, cheatgrass may play a role in the overall net carbon exchange in sage steppe communities that it has colonized (Prater et al, 2006). Sagebrush species are effective carbon sinks, with longer life cycles and larger biomass, capable of storing greater amounts of atmospheric CO2. Thus, the reduction in sagebrush density and increased colonization of cheatgrass, an annual grass with little capacity for CO2 storage, has profound implications for CO2 emissions in the surrounding landscape. The cascading results of increased CO2 include warmer mean temperatures and decreased precipitation, leading to greater propensity for fire disturbance as well as lower rates of sagebrush recovery, as recovery rates of sagebrush are negatively correlated with dry conditions in the year immediately following fire disturbance (Nelson et al, 2014).
The sage steppe landscape at Deer Flat National Wildlife Refuge (DFNWR) experienced a human-caused fire, named the Sage Fire, on July 5, 2003. Prior to the fire, the sage steppe community was already composed primarily of cheatgrass with low densities of both native grasses and sage brush (Patton, 2003). Thus, even the unburned site in the following study does not represent a sage steppe community in its pristine state, as the entire landscape had already experienced frequent fire events, degrading the community to one in transition between an invaded state to an annual dominance state. Nevertheless, post-fire recovery strategies were focused exclusively on the burned site to suppress the colonization of cheatgrass and aid in the recovery of native grasses and sagebrush. These strategies included herbicidal treatment on cheatgrass and other non-native species and drill-seeding of native and native-friendly species (Patton, 2003). The objective of this research is to determine the efficacy of the post-fire recovery strategies at the Sage Fire by comparing unburned sites to burned/treated sites and analyzing differences between non-native understory, native bunchgrass, and sagebrush/overstory density between sites. According to Nelson et al. (2014), sagebrush recovery is a slow process, taking anywhere from 15-100 years, with greater cool-season precipitation accelerating sagebrush recovery rates, particularly in the season immediately following disturbance. Typical climatic patterns at DFNWR include wetter winters and somewhat arid summers (Patton, 2003).
Therefore, with the combination of 17 years having elapsed and the likelihood of favorable, high precipitation conditions in the cool season immediately following fire disturbance, we predict that recovery efforts will demonstrate a moderate degree of success, effectively increasing sagebrush density to comparable levels of those found in the unburned site. To accept this hypothesis, we should expect to find no significant difference in sagebrush density between burned and unburned sites. If recovery strategies have not been successful, we should expect to see significantly reduced sagebrush density in the burned/treated site when compared to the unburned site.
Furthermore, we hypothesize that aggressive herbicidal treatment and drill-seeding efforts will yield significantly reduced cheatgrass and other non-native understory cover and increased native bunchgrass cover in the treated sites when compared to the unburned/untreated sites. No difference between cheatgrass, other non-native species, and bunchgrasses between sites indicate limited success. Considering the fact that even the unburned site was in an invaded state, the desired goal of recovery should be to significantly reduce non-native understory and increase native grass cover even when compared to the reference (unburned) site. Finally, an increased abundance of cheatgrass and other non-native species with a decreased abundance of native bunchgrasses when compared to the unburned site would indicate that recovery strategies have not been effective at mitigating invasion of non-native species or recovering bunchgrasses in the burned site.
MATERIALS AND METHODS
We established two treatment groups, denoted as unburned/untreated and burned/treated sites at DFNWR, each consisting of eighteen 50 m long randomly placed transects (Fig. 1). Understory density, including estimates of bunchgrass, cheatgrass, and other non-native species cover, was estimated using a 1 m2 quadrat every 5 m along each transect, accounting for a total of 198 quadrat data points per treatment group (Daubenmire, 1959). However, due to missing data points, the burned and unburned groups had respective sample sizes of 191 and 192. Estimation of understory density was calculated with a modified Daubenmire cover class method on a scale from 0-6.
(Table 1)
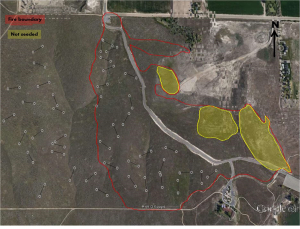
Table 1: Daubenmire Cover Class Metrics | |||||||
Cover Class | 0 | 1 | 2 | 3 | 4 | 5 | 6 |
Percent Range | 0% | 1-5% | 5-25% | 25-50% | 50-75% | 75-95% | 95-100% |
Percentage estimates were each converted to their midpoints, and the resulting data were evaluated with descriptive statistics and histograms to assess a normal distribution of the data. We calculated the occurrence of each understory component using the COUNTIF- function in Excel. Data across treatments were analyzed with three two-sample t-tests assuming unequal variances comparing differences in cover percentage between unburned and burned sites for cheatgrass, native bunchgrasses, and other non-native species. For overstory density, points were established every 10 m along the previously established transects for a total of 108 data points per treatment group. Due to missing data points, the actual sample sizes were 106 and 108 for burned and unburned treatment groups, respectively. A point-center quarter method (Curtis, 1956) was utilized to estimate mean distance from each point to the nearest overstory species, usually identified as Artemesia tridentata, although four wing saltbush (Atriplex canescent) and grey rabbitbrush (Chrysanthemums nauseous) were also included for overstory density analysis. The mean distance was then converted to density measured as shrubs per 10 m2. The resulting data were then compared between treatment groups with a two-sample t-test assuming unequal variances. Additionally, cumulative mean and cumulative standard deviation were both calculated and plotted to determine reliability and consistency of the collected data.
RESULTS
Understory Analysis
Significant differences were found for all understory species measured between burned and unburned treatment groups. Analyses of bunchgrasses (t-test, p=5.5 × 10-14, df=212), cheatgrass, (t-test, p=1.8 × 10-14, df=357) and other non-native species (t-test, p=1.5 × 10-9, df=376) demonstrated statistically significant differences between treatments for foliar cover of each understory species. Mean foliar cover of native bunchgrasses for the burned sites was 11.5%, while the unburned sites had a mean foliar cover of 0.3% (Fig. 1a). Bunchgrass foliar cover was an estimated 38 times higher in burned/treated sites than in unburned/untreated sites. Mean covers of 67.4% and 45.8% were found for cheatgrass in unburned and burned sites, respectively (Fig. 1b). Cheatgrass foliar cover was approximately 1.5 times higher in unburned sites when compared to burned sites. Mean covers for other non-native species were 33.6% for unburned sites and 17.7% for burned sites (Fig. 1c). Non-native species foliar cover was 1.9 times higher in unburned sites than burned sites.
Analysis of frequency for understory species (Fig. 2) found cheatgrass occurrence between burned and unburned treatments to be nearly equivalent with respective frequencies of 98% and 99%, while occurrences of native bunchgrasses were much higher in burned/treated sites (50% frequency) when compared to unburned/untreated sites (0.5%). Finally, other non-native species frequency was somewhat lower in the burned sites (79% frequency) when compared to the unburned sites (98% frequency).
Overstory Analysis
Mean shrubs per 10 m2 in the burned sites was 0.069 compared to 0.085 shrubs per 10 m2 in the unburned sites (Fig. 3). Standard deviation was 0.16 in unburned sites and 0.11 in unburned sites. T-test analysis (p-value=0.36) did not find any statistically significant difference in overstory density between burned and unburned sites. Overstory density in the burned sites was 81% of the overstory density found in the unburned sites.
Cumulative mean and standard deviation values for overstory data collected in the burned treatment group demonstrated variability in the cumulative standard deviation values throughout the dataset, with standard deviation values exceeding mean values and minimum values overlapping values of zero (Fig. 4). In the unburned treatment group, cumulative mean and standard deviation values approached equilibrium approximately halfway through the dataset, but still contained minimum standard deviation values that overlapped zero (Fig. 5)
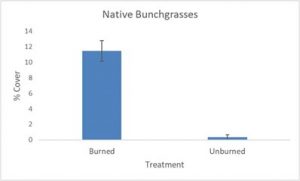
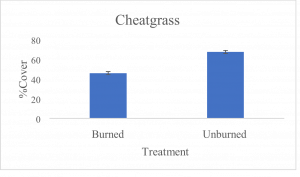
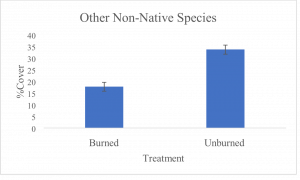
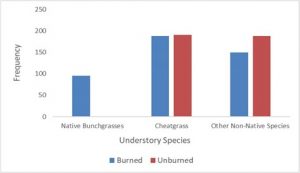
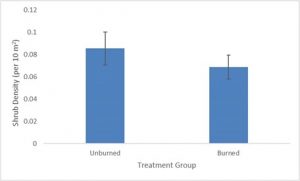
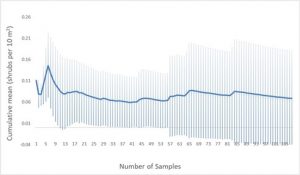
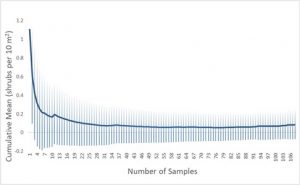
DISCUSSION
A significantly higher percentage of foliar cover for bunchgrasses in the burned treatment group, when compared to the unburned treatment group, suggests that re-seeding efforts have successfully increased the presence of bunchgrasses in the burned sites. Additionally, significantly lower cover of cheatgrass in the burned sites may suggest that herbicidal application effectively reduced the degree of cheatgrass dominance in the burned sites. However, research conducted by Lehnhoff et al. (2019), which implemented herbicidal treatments for management of cheatgrass, found that cheatgrass cover was not significantly reduced by herbicidal treatment alone. Their study showed, however, that herbidical application did increase percent cover of native bunchgrasses. Thus, it is possible that herbicidal applications at DFNWR did not effectively kill or hinder growth of cheatgrass, but facilitated growth of bunchgrasses, indirectly reducing cheatgrass cover by allowing for increased cover of bunchgrasses to outcompete cheatgrass in treated areas.
Fig. 2 demonstrates that frequency of cheatgrass was similar between treatment groups; however, Fig. 1b reflects a significant reduction in percent cover of cheatgrass in the burned site.
This suggests that cheatgrass prevalence is still a concern, although progress is being made in reducing its dominance in the burned and treated sites. According to Bates et al. (2011), fire disturbances facilitate colonization of cheatgrass particularly when those disturbances destroy existing native bunchgrasses. This infers that re-seeding of bunchgrasses appears to be an important recovery strategy to successfully reduce cheatgrass dominance post-fire disturbance. Overall, the burned/treated site had both increased bunchgrass cover and decreased non-native weed cover (including cheatgrass), suggesting that restoration efforts have made a positive impact for the burned/treated site.
The dominant understory species in both the unburned and burned sites remain to be cheatgrass, with other non-native species also accounting for a greater percent composition of understory than native bunchgrasses in both sites. Prevalence in the frequency of cheatgrass in both unburned and burned sites suggest that disturbance events have likely occurred in both sites, facilitating the colonization, and spread of cheatgrass (Bradford & Lauenroth, 2006); thus, restoration efforts (herbicidal application and planting native bunchgrasses) may be needed to reduce and control cheatgrass cover in both burned and unburned sites. Other combination strategies conducted by Lehnhoff et al. (2019), such as controlled grazing combined with herbicidal application, may be worth consideration as a strategy to significantly decrease cheatgrass frequency in both burned and unburned sites. The concern with this strategy is that while this combination of treatments successfully reduced cheatgrass cover, it did not increase native grass cover. A rotation of grazing/herbidical treatment followed by aggressive re-seeding of native bunchgrasses may be a worthwhile recovery strategy to consider at DFNWR.
While recovery metrics proposed by Germino (2017) suggest a target understory foliar cover of bunchgrasses of at least 20%, the treated area at DFNWR showed only 11.5% native bunchgrass cover. Due to repeated fire disturbances in this area, it may be unrealistic to expect Germino’s proposed recovery metrics at DFNWR unless frequency of fire events can be successfully reduced via prevention strategies. Native bunchgrasses behave as competitive, midsuccessional species following disturbance; thus, given enough time, these grasses are likely to dominate over ruderal non-native species such as cheatgrass (Hanna and Fulgham, 2015), but reclaiming dominance in sage steppe ecosystems relies on preventing frequent disturbance events, which is difficult to control.
There was no statistically significant difference of overstory density between treatments, and overstory density in burned sites was 81% of overstory density in unburned sites, which suggests that recovery efforts have made significant progress in restoring overstory density in burned sites to pre-fire conditions. Sagebrush recovery appears to be on track with expected recovery rates, with 17 years passing since the fire event and marked progress in sagebrush recovery being observed in the burned site. Observed average recovery time for sage steppe ecosystems is between 15 and 100 years (Nelson et al., 2014), which indicates that recovery is making headway on track with expected recovery rates. While the burned sites did show slightly lower density estimates, overlapping error bars derived from respective standard deviation values underscore the similarities between density values across both treatments.
Limitations to this study include the methodology utilized to collect data on the understory species as well as the sample sizes of the overstory species data. For example, a modified Daubenmire cover class method, while commonly used for ease and convenience, does not yield precise estimates of foliar cover. With large ranges within each cover class, midpoint values procured from this method of estimation may be significantly different than if another metric was used, such as measuring actual percentage estimates for each 1 m2 quadrat. Using midpoint values also skews the normality of the data, in contrast to actual percentage estimates which could be assessed for normality.
Additionally, variation in overstory estimates arose likely due to a number of potential causes. Cumulative mean and standard deviation values, particularly in the burned site (Fig. 4) indicated that more data points may have been needed to yield more accurate and reliable results for shrub density. Cumulative mean and standard deviation values in the unburned treatment group, by contrast, demonstrated a sufficient sample size for equilibrium of both metrics, (Fig. 5), but still yielded standard deviation minimum values that overlapped zero. In the future, more precise measurements of foliar cover, such as specific estimates for each quadrat, as well as larger sample sizes for shrub density, may increase reliability of the data, offering a clearer picture of the efficacy of recovery efforts at DFNWR. Variations in soil type were also not accounted for, which may significantly impact plant community structure and recovery efficacy (Gehring, et al., 2016). Slope and aspect of sites may also account for variability in plant composition due to variations in light and nutrient availability between different combinations of slopes and aspects throughout the landscape. In the future, accounting for slope and aspect as well as soil type variability throughout sampled transects may reflect more accurate differences between burned and unburned treatment groups.
Although the data indicates that recovery efforts have had a significant and positive impact on the sage steppe ecosystem in the burned/treated sites, both bunchgrass cover and sagebrush density still remain low across both treatment groups. This suggests that more than post-fire recovery strategies may be needed in order to make a difference for the many animals that depend on sage steppe ecosystems, such as the sage grouse (Centrocercus urophasianus), which depends on an abundance of sagebrush not only for habitat cover, but also for the array of ecologically connected biota that sagebrush give rise to, such as perennial native forbs and insects, which young sage grouse depend on for nutrition (Dumroese et al., 2015). Post-fire recovery strategies at DFNWR may not effectively provide sage grouse with their habitat needs. According to Ellsworth et al. (2016), sage grouse generally depend on 15-25% shrub density for nesting. While recovery efforts have shown an increase of shrub density compared to levels immediately post-fire, there is clearly more work to be done to restore the sage steppe community at DFNWR to optimal levels for its many inhabitants, in both burned and unburned sites.
Future studies on native animal populations within sage steppe ecosystems between post-fire and undisturbed treatments could offer insight on the impact that sage steppe ecosystem recovery efforts are making on these species. Focusing on the larger objective of sage steppe recovery, such as investigating whether restoration is positively impacting sage grouse and other animals dependent on these ecosystems, could help determine whether these efforts are worthwhile and effective at achieving the ultimate goals of sage steppe restoration. Furthermore, studies on the invasions of animal species following fire disturbance in sage steppe ecosystems could help inform our understanding of ecological shifts within sage steppe ecosystems beyond the scope of plant invasions.
REFERENCES
Bates, J. D., Davies, K. W., Sharp, R. N., 2011. Shrub-Steppe Early succession following juniper cutting and prescribed fire. Environmental Management 47, 468–481.
Bradford, J.B., Lauenroth, W.K., 2006. Controls over invasion of Bromus tectorum: The importance of climate, soil, disturbance, and seed availability. J Veg Sci 17, 693.
Chambers, J.C., Bradley, B.A., Brown, C.S., D’Antonio, C., Germino, M.J., Grace, J.B., Hardegree, S.P., Miller, R.F., Pyke, D.A., 2014. Resilience to stress and disturbance, and resistance to Bromus tectorum L. Invasion in cold desert shrublands of Western North America. Ecosystems 17, 360–375.
Curtis, 1956. The use of distance measure in phytosociological sampling. Ecology 37:451-460.
Daubenmire, R., 1959. A canopy-coverage method of vegetational analysis. Northwest Science 33(1): 43-64.
Dumroese, R.K., Luna, T., Richardson, B.A., Kilkenny, F.F., Runyon, J.B., 2015. Conserving and restoring habitat for Greater Sage-Grouse and other sagebrush-obligate wildlife: the crucial link of forbs and sagebrush diversity. Native Plants Journal 16, 276–299.
Ellsworth, L.M., Wrobleski, D.W., Kauffman, J.B., Reis, S.A., 2016. Ecosystem resilience is evident 17 years after fire in Wyoming big sagebrush ecosystems. Ecosphere 7.
Gehring, C.A., Hayer, M., Flores-Rentería, L., Krohn, A.F., Schwartz, E., Dijkstra, P., 2016. Cheatgrass invasion alters the abundance and composition of dark septate fungal communities in sagebrush steppe. Botany 94, 481–491.
Germino, M. 2017. What can be learned for BOP NCA from observing the Soda Fire response? Oral presentation. Morley Nelson Snake River Birds of Prey National Conservation Area Science Working Group Annual Symposium. October 30, 2017.
Hanna, S.K., Fulgham, K.O., 2015. Post-fire vegetation dynamics of a sagebrush steppe community change significantly over time. Cal Ag 69, 36–42.
Lehnhoff, E.A., Rew, L.J., Mangold, J.M., Seipel, T., Ragen, D., 2019. Integrated Management of Cheatgrass (Bromus tectorum) with Sheep Grazing and Herbicide. Agronomy 9, 315.
Nelson, Z.J., Weisberg, P.J., Kitchen, S.G., 2014. Influence of climate and environment on postfire recovery of mountain big sagebrush. Int. J. Wildland Fire 23, 131.
Olson, L.E., Oakleaf, R.J., Squires, J.R., Wallace, Z.P., Kennedy, P.L., 2015. Nesting pair density and abundance of ferruginous hawks (Buteo regalis) and golden eagles (Aquila chrysaetos) from aerial surveys in Wyoming. Journal of Raptor Research 49, 400–412.
Prater, M.R., Obrist, D., Arnone, J.A., DeLucia, E.H., 2006. Net carbon exchange and evapotranspiration in postfire and intact sagebrush communities in the Great Basin. Oecologia 146, 595–607.
Patton, W. 2003. Sage fire burned area emergency stabilization and rehabilitation (ESR) plan. U.S. Fish and Wildlife Service, Deer Flat National Wildlife Refuge.
Whiting, J.C., Doering, B., Wright, G., Englestead, D.K., Frye, J.A., Stefanic, T., Sewall, B.J., 2018. Long-term bat abundance in sagebrush steppe. Sci Rep 8, 12288.